2019 Activity Report for Mission 3: Sustainable Space Environments for Humankind
更新日: 2020/06/12
Research 1: Simulation study on substorms
Principal Investigator (PI): Yusuke Ebihara (RISH, Kyoto University)
Research collaborator(s): Takashi Tanaka (Kyushu University), Naoki Kamiyoshikawa (RISH, Kyoto University)
When an auroral breakup occurs, a large amount of energy (>1011 W) is consumed in the upper atmosphere in the polar region. The auroral breakup is regarded as a transient phenomenon, so that the magnitude of the auroral breakup is thought to be difficult. Using large-scale computer simulation with various solar wind parameters, we found that the maximum magnitude of the substorm (in terms of auroral electrojet) is roughly proportional to the incident energy through the magnetopause. About 33-88% of the incident energy originates from the solar wind kinetic energy. Energy flow and conversion from the solar wind to the Earth are clearly identified.
Publication
- Ebihara, Y., Mechanism of auroral breakup, Japanese Journal of Multiphase Flow, 33, 3, 267-274, doi:10.3811/jjmf.2019.T012, 2019.
- Ebihara, Y., and T. Tanaka, Evolution of auroral substorm as viewed from MHD simulations: Dynamics, energy transfer and energy conversion, Reviews of Modern Plasma Physics, 4:2, doi:10.1007/s41614-019-0037-x, 2020.
Research 2: Study on dynamic variation of relativistic electron fluxes in the radiation belts
Principal Investigator (PI): Yoshiharu Omura (RISH, Kyoto University)
Research collaborator: Yikai Hsieh (RISH, Kyoto University)
We have found that relativistic electrons in the Earth’s radiation belts are generated through nonlinear interaction with whistler-mode chorus emissions and that these electrons are precipitated by EMIC-triggered emissions. Nonlinear wave processes are essential, and they are clarified by test particle simulations. Using results of test particle simulations of electrons interacting with whistler mode chorus emissions, we numerically obtain Green’s functions to model evolution of the electron distribution function after all of the possible interactions with the waves. In both wave models with and without subpackets, electrons undergoing the cyclotron resonance with the waves are efficiently accelerated by nonlinear wave trapping. Since the strong modulation of the wave amplitude (in the case of the waves with subpackets) affects dynamics of resonant electrons, the electrons are detrapped from the wave potential or entrapped into it more frequently than those interacting with the waves without subpackets. As a result of interaction with the waves with subpackets, the number of electrons undergoing the acceleration is increased, while the acceleration efficiency in energy is decreased because of the shorter interaction time. Modifying the numerical Green’s function method with the simplified model of chorus waves uniform in longitude (Omura et al., 2015, https://doi.org/10.1002/2015JA021563), we compute the formation process of the outer radiation belt electron fluxes induced by the interaction with the chorus waves localized in longitude occurring for a time scale of 1 hr. The formation of MeV electron fluxes is characterized by large acceleration rates and butterfly pitch angle distributions, which are found in satellite observation results.

Formation of radiation belt due to electron acceleration by chorus emissions localized in longitudes
Research 3: Development of miniaturized plasma wave instruments for monitoring space electromagnetic environments
Principal Investigator (PI): Hirotsugu Kojima (RISH, Kyoto University)
Research collaborators: Motoyuki Kikukawa (Graduate school of engineering, Kyoto university), Kazushi Asamura (JAXA)
Wave-particle interaction is a basic process of energy transportation in space plasmas. Since space plasmas are intrinsically colisionless, kinetic energies of plasma particles are exchanged through wave-particle interactions. One of key issues in understanding space electromagnetic environments through satellite observations is to directly measure wave-particle interactions. Wave-particle interaction analyzer or (WPIA) is a unique method for quantitatively evaluating wave-particle interactions in satellite observations. It has the capability to identify relative phase angles between particle velocity vectors and wave vectors, which are essential in pursuing energy flows. However, the WPIA requires a lot of resources in its mass, size and power consumption. That does not fit capacities of small- or nano-satellites. To meet the resources of small- or nano-satellites, the present study attempts to miniaturize the WPIA circuit using the ASIC technology. In FY2019, the miniaturization of particle detecting circuits have been made. The figure shows the layout inside the designed chip. It contains a series of circuits that pick up current pulses from particle sensors and amplify the signal as the voltage output. The size of the circuit is 0.21mm x 0.57mm for one channel. The output of this circuit is fed to a plasma wave receiver to compare with waveform data. The result well shows the feasibility of so-called one-chip WPIA. The further detailed design of the chip will be made in the future.
Research 4: Application research into new materials for space missions
Principal Investigator (PI): Yoshikatsu Ueda (RISH, Kyoto University)
Research collaborator(s): Yomei Tokuda, Liao Zhenghao (Tongji University, China), Vishnu Thonglek, Rattanaporn Norarat(Rajamangala University of Technology Lanna, Thailand)
We conduct basic and application research on fine bubble technology as a new material expected for future space mission. We also have been collaborating with internal/international university as interdisciplinary mission theme.
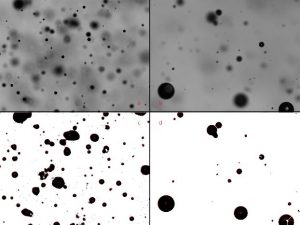
High-speed image of microbubble generation with/without UFB (a: original photo of ejected water with UFB; b: original photo of ejected water without UFB; c: photo a after analyze particles; d: photo b after analyze particles)
Research 5: Microstructural analysis of wood-based, diamond-like carbon for improved resistance against atomic oxygen
Principal Investigator (PI): Toshimitsu Hata (RISH, Kyoto University)
Research collaborator(s): Hirotugu Kojima, Yuki Tobimatsu (RISH, Kyoto University)
Critical and rapid deterioration of the outer surface of a spacecraft is known to occur in low earth orbit (LEO) at altitudes of 200 to 700 km through interaction with atomic oxygen (AO) in complex space environments. Lignin obtained from beech, Japanese cedar and rice were prepared in order to study the availability of woody carbon materials in the space. Transmission electron microscopy (TEM) and image analysis was applied to reveal a quantitative structural change in such lignin-based carbon. The spacings of carbon lattices of beech, Japanese cedar and rice resulted in 0.44, 0.44, and 0.32 nm, respectively, by carbonization at 900ºC. The percentage of each interplanar spacing was 41, 59, and 22% as compared to the control before carbonization. The lignin-derived carbon material, which has potential of protecting a spacecraft by adsorbing AO into micro pores.